How a Heat Pump Actually Works — With Physics! And Charts!
Curious about the actual physics of how a heat pump works? Learn about it here.
There are roughly a gazillion articles scattered across the internet that describe, in very basic terms, how a heat pump works. The general idea is:
- A heat exchanger transfers heat from the hot refrigerant into the less-hot home.
- The refrigerant is decompressed, which makes it very cold.
- Another heat exchanger transfers heat into the cold refrigerant from the less-cold outdoor air.
- Refrigerant is compressed, which makes it hot.
- Start over again at #1 and repeat the cycle.
And this process “moves” heat from the cold outdoors into your warm home.
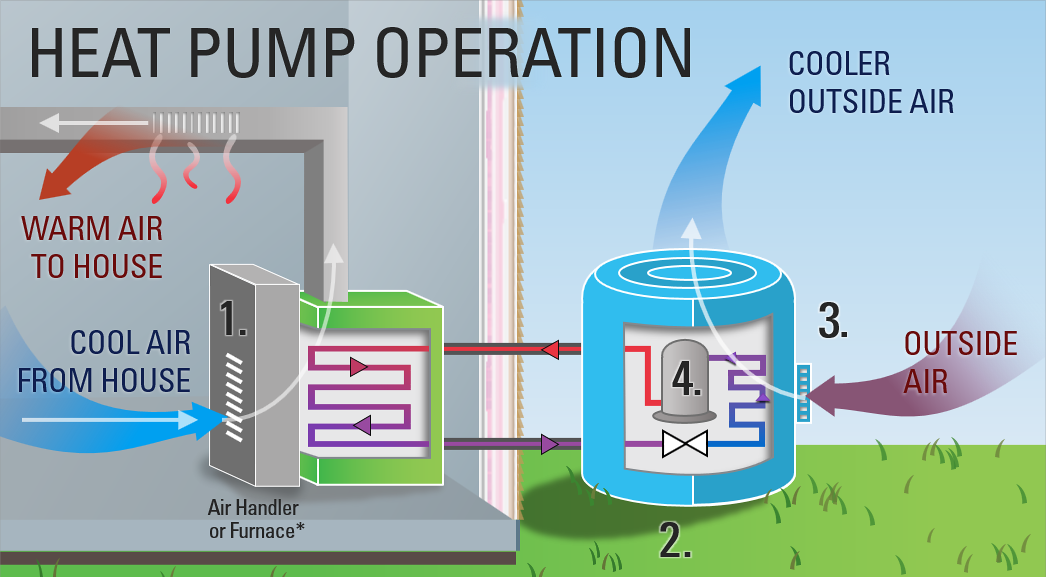
This simple explanation is a good start! But the more you learn about heat pumps, the more you realize it leaves out a lot of the details.
So let’s dig a little deeper.
Temperature vs. Heat Energy
The first key to understanding how heat pumps actually work is to understand heat energy (which physicists call enthalpy). This is the amount of energy stored in a particular amount of a particular substance as heat, relative to absolute zero. Heat energy is expressed as energy per mass (BTU/lb in the US; kilojoules/kilogram everywhere else).
Heat energy is very closely related to temperature. As you add heat energy to (or remove heat energy from) something, its temperature increases (or decreases) correspondingly. However, there is an important exception to this rule: as a substance changes phases (e.g. from liquid to gas), adding or removing heat energy doesn’t change the temperature at all. Instead, adding heat energy evaporates more liquid into gas, and removing it condenses more gas into liquid.
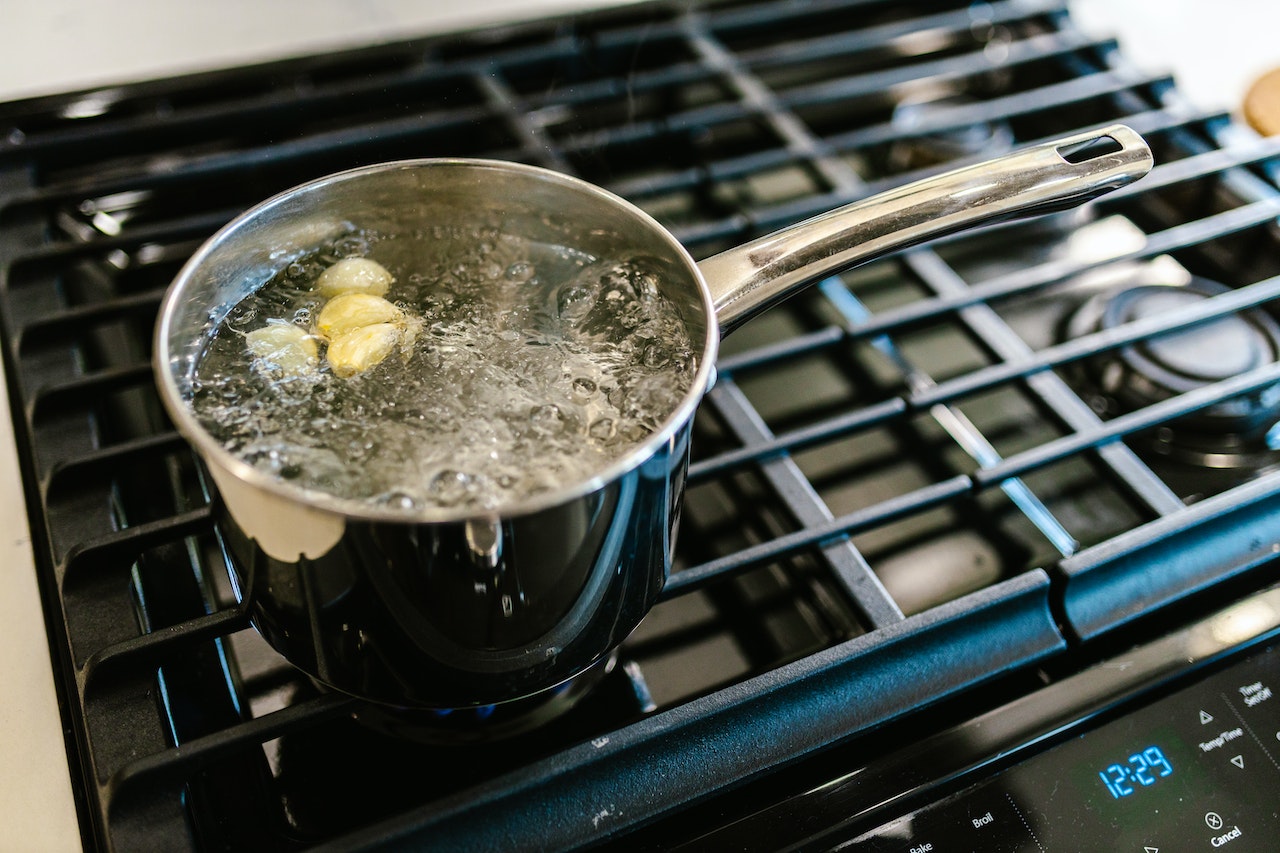
We’ve all seen this phenomenon in the real world when we boil a pot of water. The stove continually adds heat energy to the water, and the water gets steadily hotter – until it reaches its evaporation temperature of 212°F, at which point it never gets any hotter. Instead, the water starts evaporating away into steam.
The chart below shows the relationship between heat energy and temperature for today’s most common refrigerant, R-410a. You can see that as heat energy is added to the liquid refrigerant, the temperature first increases, then plateaus (at the evaporation point), then increases again once it has been fully evaporated into gas.
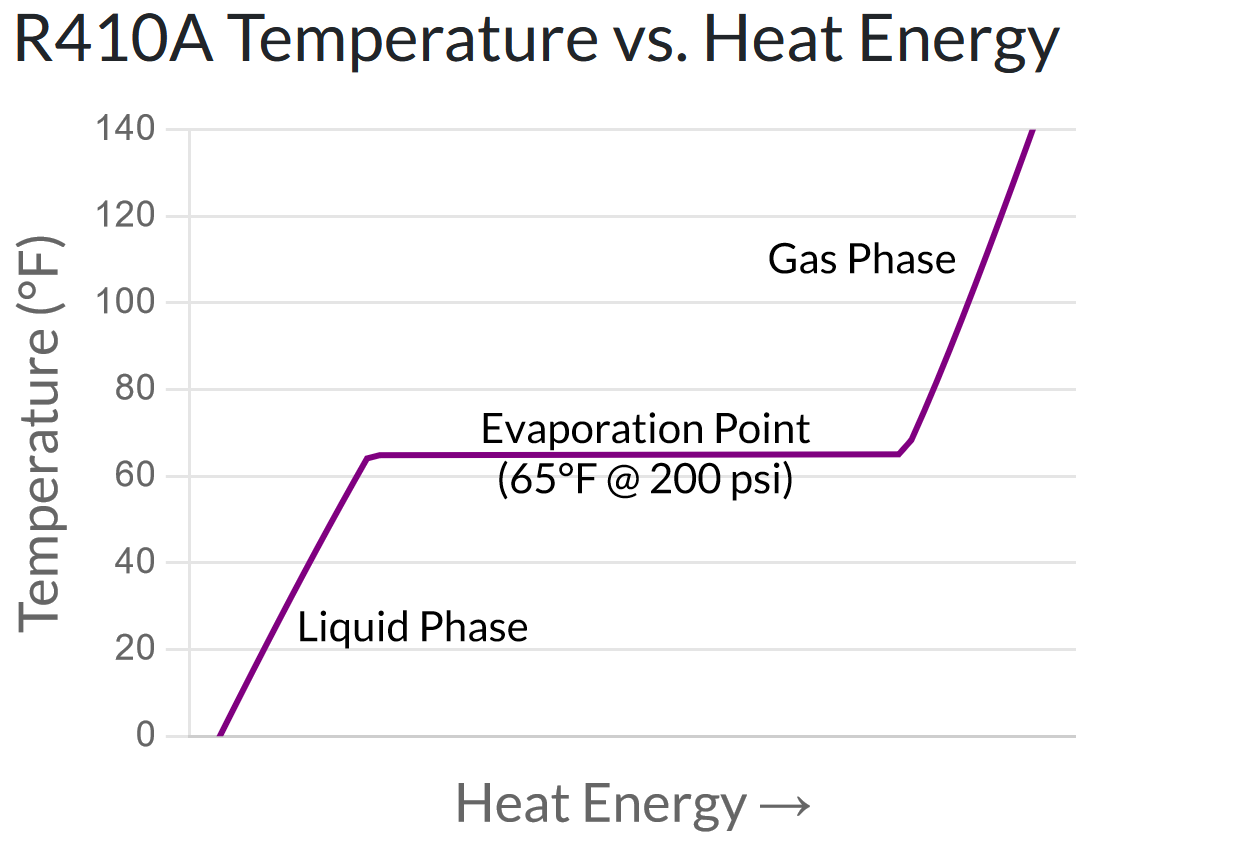
Pressure and Evaporation Temperature
The interesting thing about evaporation temperature is that it changes based on pressure. Higher pressures increase the evaporation temperature, and lower pressures decrease it.
To bring this back to our everyday example of boiling water, this is why boiling foods takes longer at high altitude – the air pressure is lower, so the water is not as hot as it boils. Similarly, pressure cookers work faster than regular boiling because the pressurized water boils at a higher temperature.
The chart below shows the same temperature vs. heat energy relationship as the previous one – but for three different pressures in the operating range of a heat pump. You can see that the higher the pressure, the higher the temperature can be increased before it plateaus at the evaporation point.
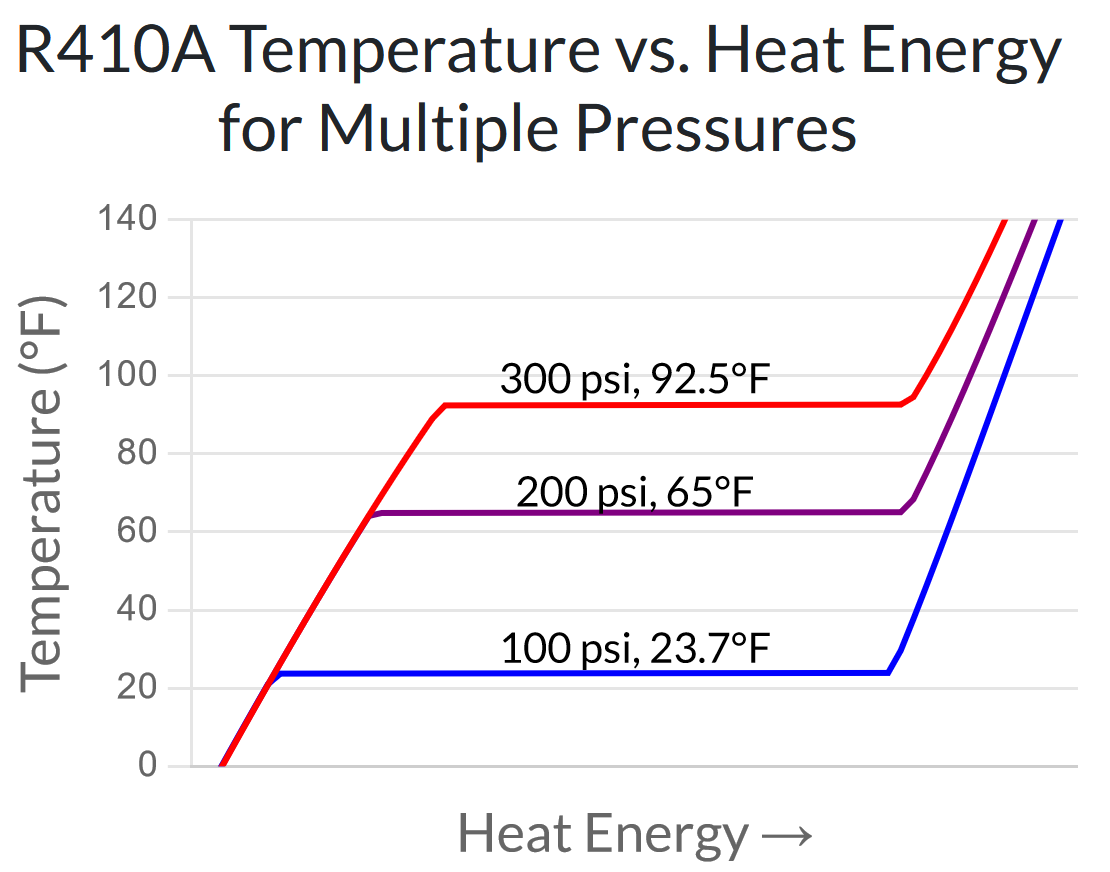
Heat pumps take advantage of this behavior. They compress the refrigerant to a level where its evaporation temperature is warmer than the inside air, so heat is transferred out of the heat pump and into the home. Then they decompress the refrigerant to a level where its evaporation temperature is colder than the outside air, so heat is transferred out of the outdoor air and into the heat pump.
Pressure and Heat Energy
When it comes to heat pumps, it makes more sense to think about the relationship between pressure and heat energy. After all, these are the two variables we directly control in the heat pump cycle. What we want to understand is how increasing/decreasing pressure and adding/subtracting heat energy affects the temperature.
The chart below shows the pressure vs. heat energy relationship of R410a at a constant temperature of 60°F.
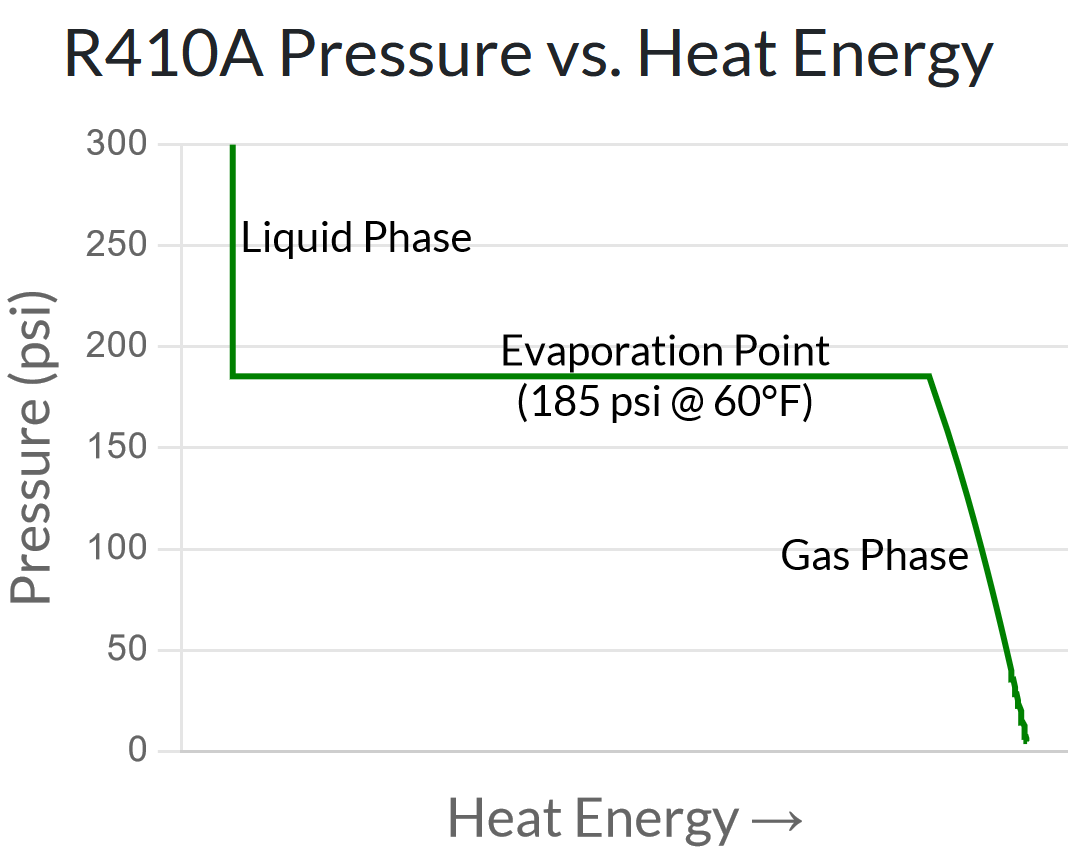
- At low heat energy, in the liquid phase, the line is nearly vertical. In other words, changing the pressure of a liquid does not affect the temperature, only the amount heat energy does.
- At medium heat energy, during the gas/liquid transition, the line is horizontal – meaning adding/removing heat energy during the evaporation process does not affect the temperature, but changing the pressure does.
- At higher heat energy, in the gas phase, the line tapers off diagonally – meaning that both pressure and heat energy affect the temperature of a gas.
If we show this same chart for every temperature increment of 20°F, we can start to see the bigger picture.
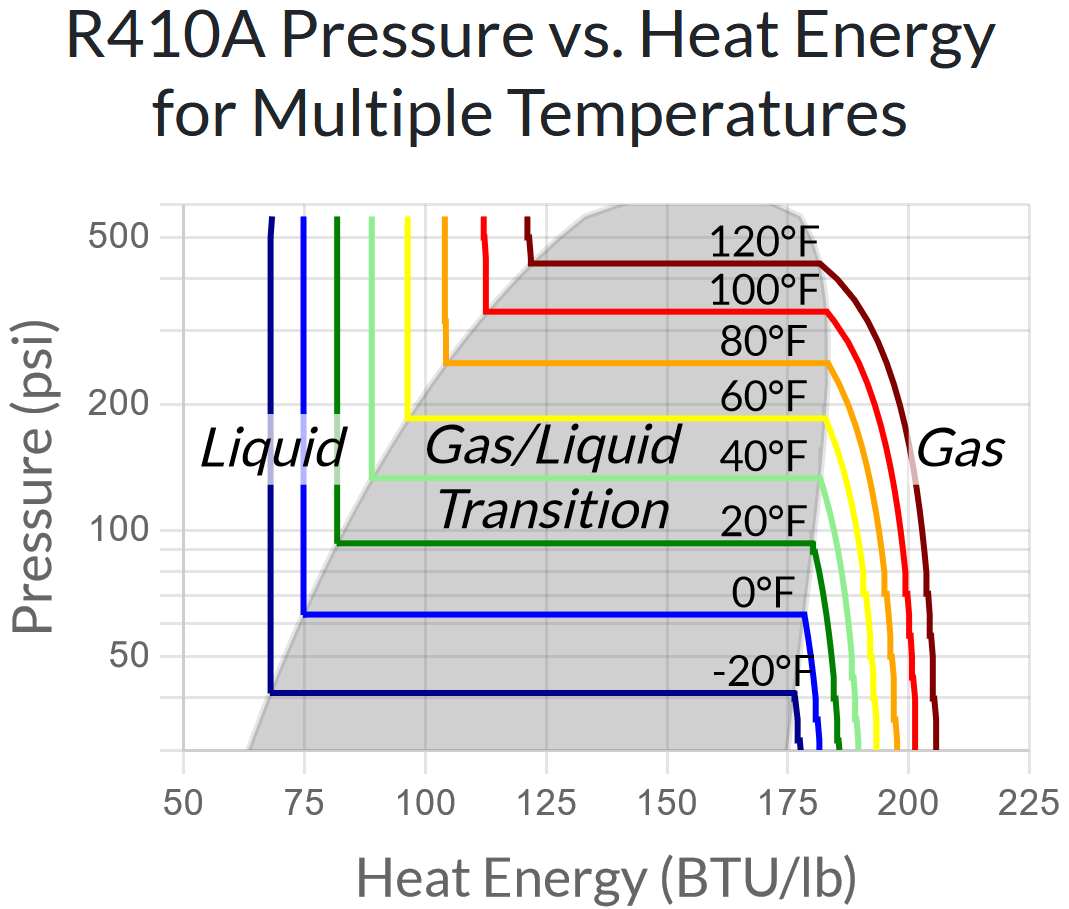
(By the way – notice that the y-axis of the chart above is logarithmic rather than linear. This is because for fluid pressure changes, the ratio of the pressures involved is more relevant than the difference. The logarithmic y-axis makes equal ratios show as equal vertical distances.)
The Heat Pump Cycle
This pressure/heat energy chart lets us sketch out the desired behavior for our heat pump. Suppose we want to run our cold heat exchanger (outdoors) at 20°F and our hot heat exchanger (indoors) at 100°F. This means we want our low and high pressures to be 93 and 333 psi, respectively. So our refrigeration cycle looks something like the following:
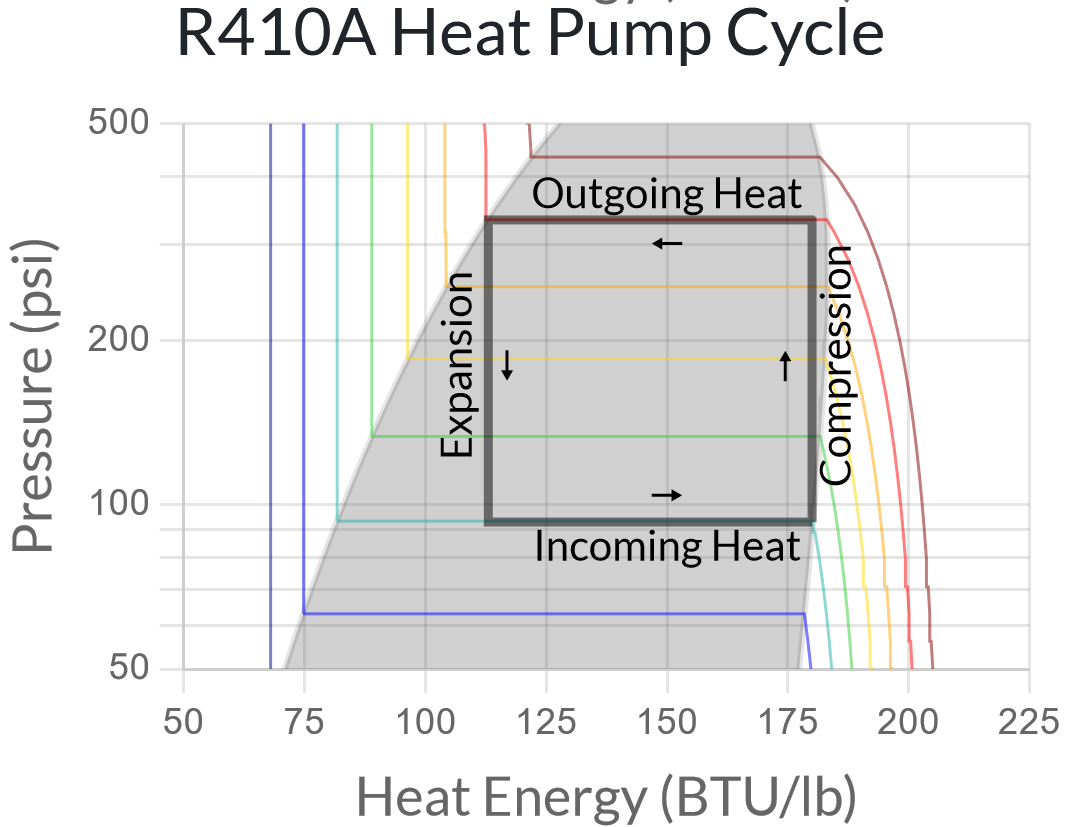
But we’re missing one important piece – compressing the refrigerant requires energy! And this energy, which is consumed by the compressor, ends up stored as heat energy in the high-pressure refrigerant.
The exact amount of energy in this process depends on the entropy of the refrigerant before it is compressed. Entropy is a weird concept that virtually nobody truly understands, but the key things to know are a) compressing a gas keeps its entropy constant, and b) doing so requires increasing the heat energy. The following chart adds constant entropy curves to the gas region of our chart (as dotted lines) so we can what this looks like:
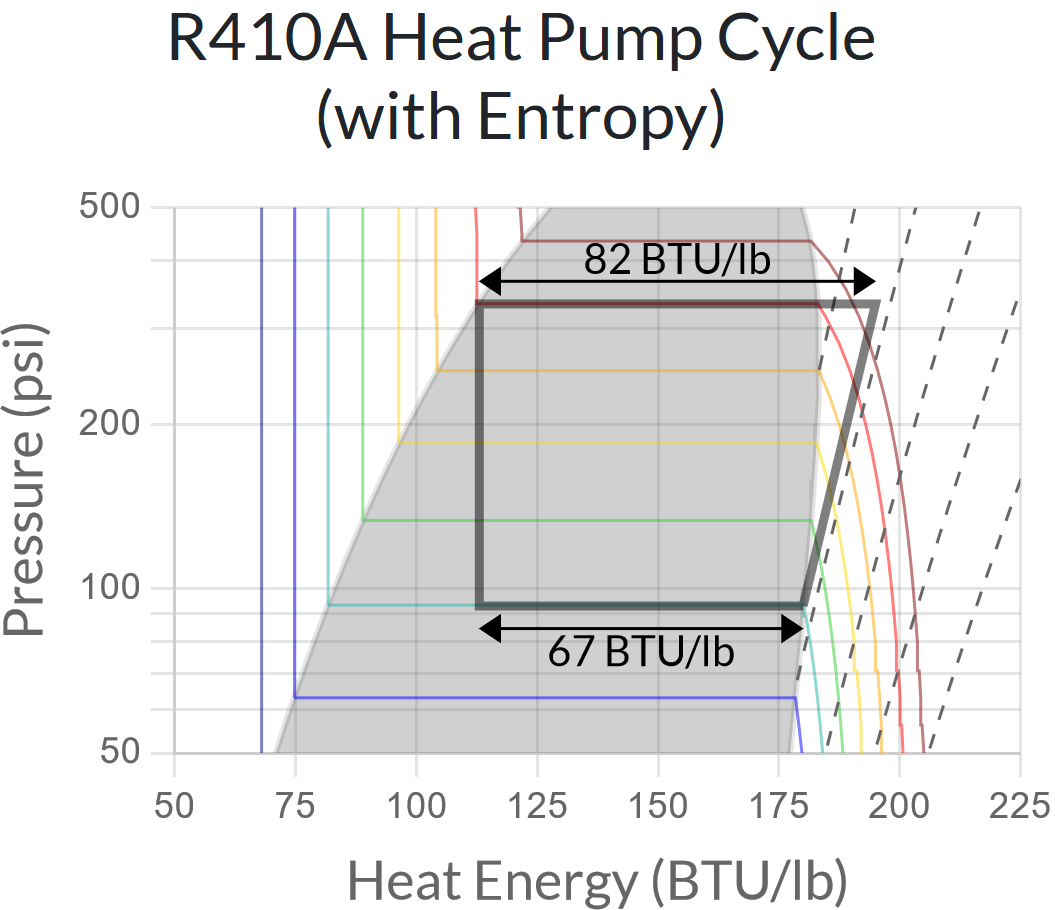
This chart tells us basically everything we are interested in knowing! The horizontal line at the top (82 BTU/lb) corresponds to our heating output power (per unit of mass flow rate through the system), the horizontal line at the bottom (67 BTU/lb) corresponds to the heat we are extracting from the outside air, and the difference (which is also the horizontal distance of the diagonal line on the right) corresponds (15 BTU/lb) to the electrical power required for the compressor. The heating efficiency (Coefficient of Performance, or COP) is the ratio of heating power to compressor electrical power.
So in this example, our heating COP is 82 ÷ 15 = 5.47
If we want to know the actual heating or cooling power in BTU/hr, we also need to know the compressor’s mass flow rate – which varies wildly by the size of the system. A typical residential system might have a mass flow rate of 500 lbs/hr, for a heating output of 82 × 500 = 41,000 BTU/hr
Practical Considerations
The heat pump cycle in the diagram above is not very realistic. In the real world,
- Compressors are not perfectly efficient; they use 30-40% more electrical energy than required, which ends up as extra heat energy in the hot refrigerant gas.
- Refrigerant gas entering the compressor is heated ~10°F above its evaporation temperature to ensure it is fully evaporated (this is called superheat).
- Refrigerant liquid entering the expansion valve is cooled ~10°F below its evaporation temperature to ensure it is fully condensed (this is called subcooling).
And of course, refrigerant in heat exchangers needs to have a meaningful temperature difference between the evaporation temperature and the surrounding air – generally around 20°F for the outdoor heat exchanger and 40°F for the indoor one. (The indoor heat exchanger has lower airflow so it needs a higher temperature difference.)
So in practice, a heat pump might use the following cycle to warm a home when it’s 30°F outside:
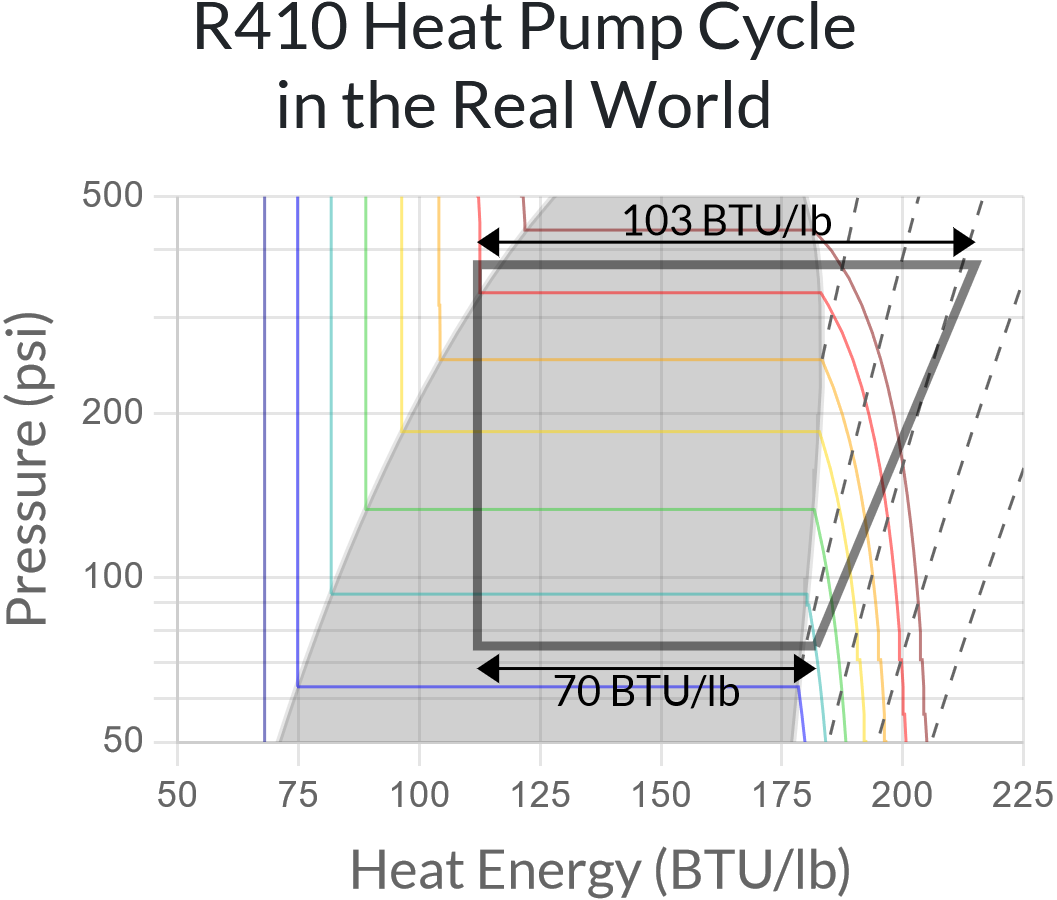
The COP in this case is 3.12, which is quite close to what real-world heat pumps are able to achieve in these conditions.
Wrapping Things Up
Hopefully this article has helped you understand how heat pumps actually work.
There’s plenty more to learn on this topic – high-end heat pumps today use all sorts of tricks to modify the heat pump cycle, like flash gas injection and intercooling. And future heat pumps will use new refrigerants that are more climate-friendly than R410a. (And here at 2040 Energy, our heat pumps for radiators are very different indeed, because they use a transcritical cycle and ejector energy recovery.)
If you’re interested in digging deeper, you can find the pressure/enthalpy data for many different refrigerants with a quick Google search. One good resource is here at The Engineering Mindset. If you are a coder, you can also use get the data from the very handy CoolProp library.
Thanks for reading! Let me know in the comments below if you have questions on this article, or what you’d like to see covered in the future.
Next: Heat Pump Startup 2040 Energy Receives Grant to Support Field Testing of Unique Heating Technology.
About 2040 Energy
We are building RenewaBoiler — the first cold-climate heat pump designed for hot water radiators. Product release is targeted for 2024.
To follow our progress, sign up for email updates below. (We promise to respect your inbox!)